· 7 min read
Shipping hydrogen on marine tankers is a somewhat hot topic these days. The EU currently thinks it is going to massively expand the role of hydrogen in its economy, especially for energy, and as a result wants to get it from other countries. Japan similarly thinks that hydrogen will displace its natural gas and coal imports, and has already received its first tiny shipment of liquified hydrogen. Fossil fuel majors are working hard to make a hydrogen economy a thing as they know that the vast majority of new hydrogen would have to come from natural gas and coal, turning their increasingly likely to be stranded assets into much more expensive hydrogen. Countries like Namibia are creating strategies to become green hydrogen superpowers, and it will take marine shipping to get it to market.
And it’s with Namibia that this assessment starts. How comparable is shipping hydrogen to other fuels? How much energy will be able to be carried on a ship in the form of hydrogen? What will it cost? These are important questions as the marine shipping industry seeks a product to replace the coal, gas and oil that constitutes 40% of all deepwater shipping, and as countries consider how they will replace the energy they currently get via ships and pipelines.
Liquid natural gas (LNG) is the best comparison, as it requires liquification, a freezing process which consumes about 10% of the amount of energy embodied in the LNG and is the closest variant of oceanic shipping to the requirements for hydrogen. A Wärtsilä-powered Q-Max ship, the biggest LNG tanker I’m aware of, carries 266,000 cubic meters of LNG. The liquified gas is cheap, with average delivered import prices in the US of $109 per cubic meter of LNG, or about $0.18 per cubic meter of natural gas, although prices are obviously spiking in late 2021 and early 2022 around the world. Those 266,000 cubic meters amounted to about $29 million USD in value before the recent spikes.
The first thing to know is that while hydrogen is energy-dense by mass, it isn’t energy-dense by volume. Assuming the same-sized ship, the delivered BTUs of energy would be about 27% of the LNG. This is because even when liquified, hydrogen has less energy by volume than LNG, but also because liquifying hydrogen takes about 33% of the energy in the liquified hydrogen, as opposed to the 10% required for LNG. Different gases, different temperatures required for liquification. Liquid hydrogen makes a lot of sense for rockets going to orbit, but its questionable economically for anything less demanding.
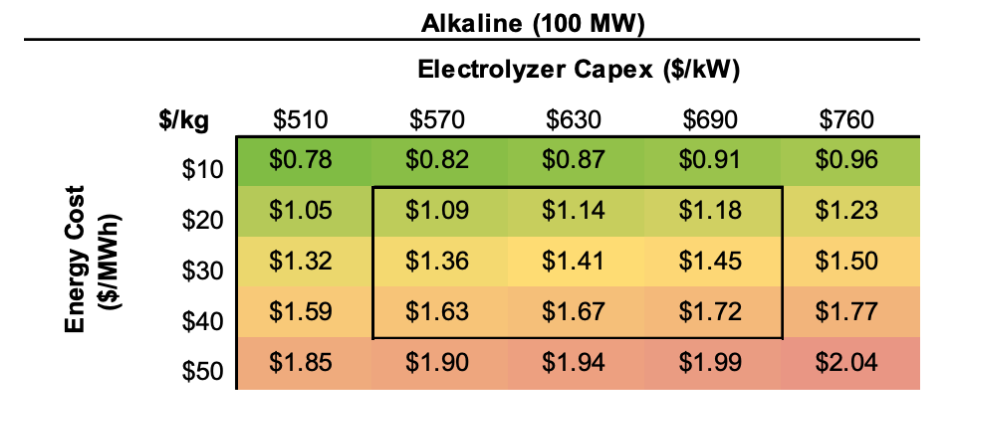
The second problem is that hydrogen by itself is expensive. The absolute best case from Lazard’s hydrogen LCOE, with dirt cheap electrolyzers, $10 per MWh electricity and 100% capacity factors, gives a cost for hydrogen of about $0.78 per kilogram at the point of production, and that excludes a large number of capital costs. A cubic meter of liquified hydrogen masses 71 kg. That means that just the absolutely best case cost of the liquified hydrogen, excluding the energy costs of liquification or getting it into the ship, would be 1.9 times as high as the delivered price of LNG. And that delivered price includes extended ocean journeys with daily LNG ship charter costs averaging of $150,000 per day, currently peaking over $200,000 per day, with a high of $350,000 per day in January 2021. There’s a bit more on the cost of a cubic meter of liquid hydrogen for liquification, but as we are pretending that electricity will cost $10 per MWh, it’s not very much, about another dollar per cubic meter.
Currently, the average for trip durations, excluding loading and unloading periods, is around 23 days, and the pre-berthing, loading, and unloading add another 4-5 days. Calling it 28 days results in an additional cost of $4.2 million.
That brings the total costs of the delivered hydrogen to roughly $19 million for 27% of the delivered energy. One assumes that the people doing this would like to make a profit as well, so assuming 10%, the total price delivered will be in the range of $21 million for the load.
That means the price per delivered unit of energy is roughly 5 times that of liquid natural gas, in the best possible case.
More realistic numbers from Lazard’s LCOE with still low $20/MWh electricity, still high 90% capacity factors, and more realistically priced but still cheap electrolyzers, would be 40% more expensive, around 7x the price of LNG per unit of energy. Doing the math with the numbers that most favor hydrogen shows how starkly bad the economics of using it for energy really are.
Of course, this is before the liquid hydrogen is converted to actually useful energy, at a maximum of 60% efficiency, which is about the same as a good combined cycle gas turbine.
What does all this mean for the actual sunshine falling in Namibia? Well, take 20% off for electrolysis, 33% off for liquification, 10% for efficiency losses for long-haul cooling and handling, and 40% off for conversion back into electricity, and the solar energy energy in Namibia turns into perhaps 29% of the energy being useful.
Of course, this doesn’t address the problem of distributing hydrogen in the country that imports it either, or the complete and utter lack of any large-scale hydrogen distribution network. 85% of hydrogen consumed globally is manufactured onsite because it’s so expensive to ship.
Delivering the same energy in the form of electricity through high-voltage direct current cables is vastly more efficient, with HVDC losses running about 3.5% per 1000 kilometers. Of course, Namibia being in southern Africa next to South Africa, it would make more sense for it to deliver the electricity there instead. Northern African solar, wind, and storage linked to fat HVDC pipes crossing the Strait of Gibraltar, crossing from Tunisia to Italy, and crossing the Bosphorus — as transmission lines already do today — makes much more economic sense. There’s a Moroccan project proposed with 20 GW of wind and solar firmed by storage, and a proposed 3,500-kilometer HVDC transmission line to the UK. Assuming the entire route is underwater, that would deliver about 88% of the generated electricity to market, not 29% — more than 3 times as much. Similarly, the Australian green hydrogen proposal originally ran HVDC transmission to Singapore, but has been distracted by the hydrogen hype and now proposes to manufacture hydrogen and ship it.
Of course, there are even less efficient ways to ship hydrogen. It could be chemically converted into a stable liquid at room temperature, and then extracted at destination, stepping on the electricity a few more times, at great loss of useful energy. It could be converted into a liquid hydrocarbon with the addition of CO2 from somewhere and upgraded to a useful plug-compatible fuel, then shipped, at only multiples of the cost of just running things off of electricity, and with the added “benefit” of air pollution.
This isn’t to say that Namibian green hydrogen can’t be useful. The country is dependent on South Africa for urea fertilizer, and could be manufacturing that itself to supply the 9% of its economy dependent on agriculture. Fertilizer needs lots of hydrogen, and once manufactured is easy to distribute, unlike hydrogen. That’s part of the reason why my projection of hydrogen demand is contrarian, with it falling over the coming decades.
Yet another straightforward analysis that accounts for the physics, the engineering, and the costs makes it clear that hydrogen is not an economically viable store of energy for our future decarbonized economy. All of the projects proposing to manufacture hydrogen where sunshine and wind are constant and cheap, and then ship it to countries that currently consume fossil fuels to replace them as an energy source fail basic tests of economics, and stack up very poorly compared to significant electrification.
Energy Voices is a democratic space presenting the thoughts and opinions of leading Energy & Sustainability writers, their opinions do not necessarily represent those of illuminem.